FUEL CELL TECHNOLOGIES
Development of next-generation proton-conducting fuel cells (PCFC) and novel electrolyte materials
In our lab, we are working on developing fuel cells that incorporate inorganic protonic conductors as the electrolyte material, ultimately aiming to design and propose novel energy systems that integrate these technologies.
Currently, the fuel cells with the highest power generation efficiencies are solid oxide fuel cells (SOFC) that use oxide ions as the charge carriers. In our lab, we aim to further improve efficiency using proton-conducting ceramic fuel cells (PCFC), which replace the oxide-ion-conducting electrolytes with proton-conducting electrolytes that have a perovskite structure (ABO3). These perovskite materials exhibit maximum protonic conductivity at 400-600˚C, lower than the 800-1000˚C temperature regime that conventional SOFCs are operated at. Such a reduction in operation temperature would enable smaller-scale energy systems that are better suited for decentralized generation. In addition, PCFCs generate water vapor at the air electrode rather than at the hydrogen electrode, resulting in higher fuel utilization and a subsequent improvement in cell voltage. We aim to exploit these advantageous characteristics of PCFCs by developing new electrolyte materials and catalytically active electrode materials, and by deepening our understanding of the electrode reaction mechanisms.
We have focused on developing novel proton-conducting solid electrolyte materials and on transforming these materials into thin-film electrolytes. Specifically, we are investigating the transport properties of charge carriers in these materials and at the interfaces, and using the insight gained from these studies to better control the transport properties and thereby improve the performance of actual devices. In recent years, we used the non-perovskite lanthanum-based proton-conducting oxide La28 – xW4+xO54+3x/2 (LWO), in hopes of addressing the issue of leakage current that occurred when using conventional perovskite materials. We successfully demonstrated that LWO can suppress hole conductivity and enable higher power generation efficiency than other protonic conductors.
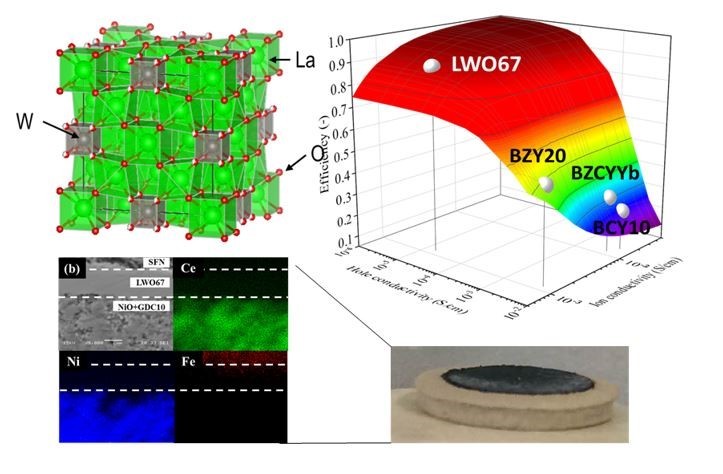
Relevant Equipment
Pulsed Laser Deposition (PLD)
We use the PLD to deposit thin layers of solid oxide thin films. A pulsed laser is directed towards a sintered target of the desired composition, to produce a plume that is deposited onto the substrate placed above. Thin-film electrolytes that are several hundred nm to several μm thick can be formed.
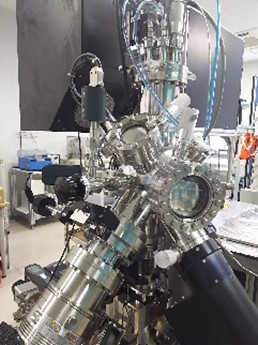
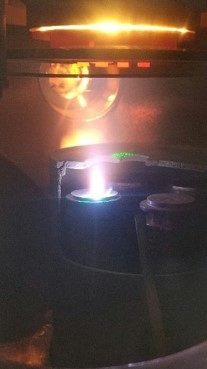
Thin-film surface characterization equipment (controlled atmosphere SPM-KFM)
We use SPM-KFM to measure the potential distribution and transport properties, for example of the interfaces of layered thin-films deposited via PLD. Controlled-atmosphere scanning probe microscopy (SPM) and atomic force microscopy (AFM) are used to observe surface morphology, and Kelvin probe force microscopy (KFM) is used to measure surface potentials.
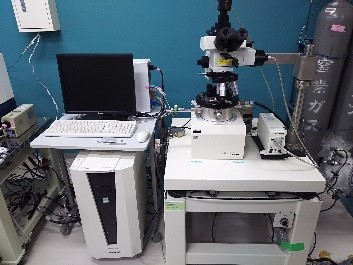
Fuel cell power generation equipment
The power generation performance of fabricated fuel cells is tested using this double-chamber generation equipment.
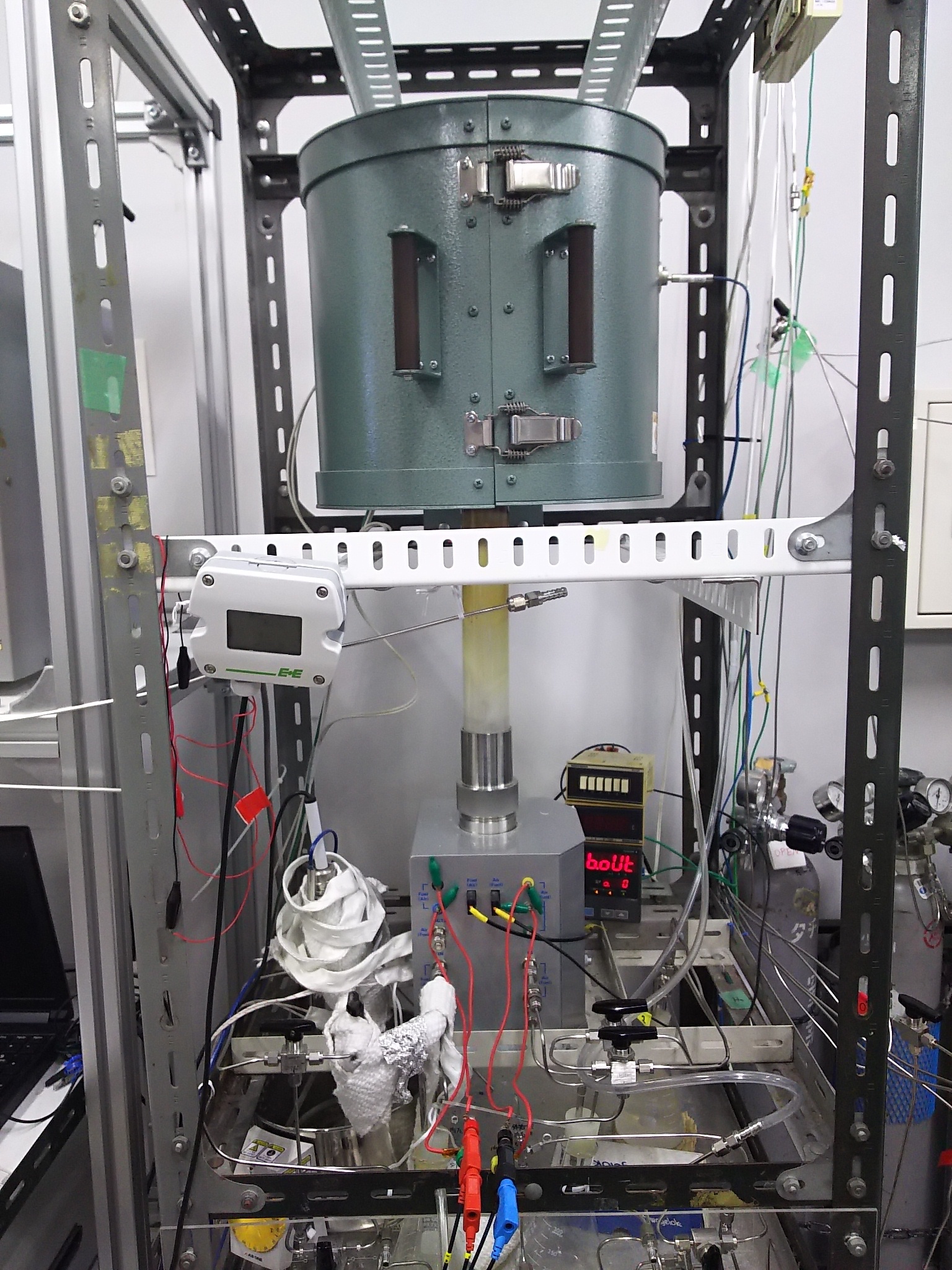